Batteries for Electric Transportation: Get the Chemistry Right
Posted on: in Blog
Quoting analyst James Frith from BNEF on Twitter, “the global electrification revolution would not be possible without batteries”. In line with the Paris Agreement- where nearly 200 countries signed an international treaty to combat climate change - the push for battery powered vehicles, from PHEV to BEV, is strongly underway. But new types of batteries are constantly being developed. This blog describes a short history of battery technologies and stresses that “It Is All About The Chemistry”!
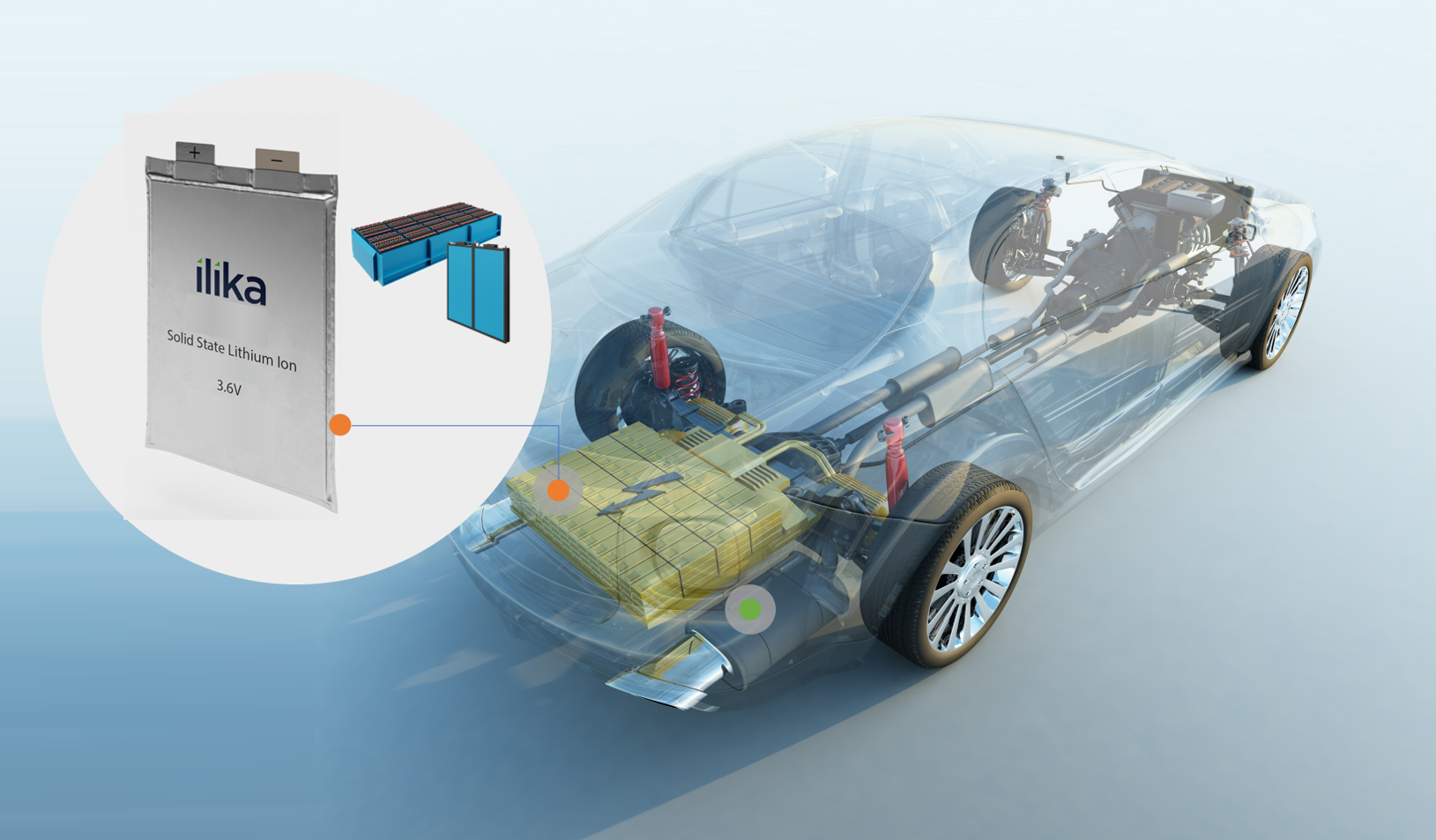
Of course, all Internal Combustion Engine vehicles already have a battery, typically with lead acid chemistry, which powers the ignition system to start the engine and support accessories like lights, indicators and the car warning system. Despite their low price these batteries were never seriously considered as a source of power to assist the vehicle’s propulsion due to their low capacity, but are still used as auxiliary batteries. NiMH chemistry batteries, which have double the energy density of lead acid batteries, were integrated in early PHEVs and have been predominant in micro hybrid vehicles. As the technology is now mature, little improvement in performance will be expected in the future and in terms of cost, the rapid decrease in price of the next technology that came along, lithium-ion, makes NiMH unlikely to be able to compete. Other Ni-based chemistries were strong for a while, such as NiCd, but it is now only limited to industrial applications that require high temperature performance or resistance to mechanical abuse.
John Goodenough, Stan Whittingham and Akira Yoshino received the Nobel Prize in Chemistry in 2019 for the development of lithium-ion batteries (LIB) and it is clear to all that this rechargeable chemistry has taken over the world, from secondary coin cells to cylindrical batteries and pouch cells. The global situation around the oil crisis of the 70s was the foundation for their research into an efficient energy storage solution that could indeed replace petrol and gas in many areas of transportation and consumer electronics. Lithium ion chemistry relies on the efficient transfer of Li ions from the cathode to the anode upon charge, and reversibly upon discharge; at the cathode, materials need to be stable upon Li intercalation for many charge/discharge cycles, which works very well with lithium transition metal oxides like olivine LiFePO4(LFP), spinel LiMn2O4or layered rock salt Li-Mn-Ni-Co-O (NMC, various stoichiometries). Various chemistries have their own advantages, such as safety and cost for LFP and energy density for NMC. Despite the many advantages of LIB being clear (for example great cycle life and rapidly decreasing costs) and the technology being the battery type of choice in current electric vehicles, the technology is also stuck in everyone’s subconscious in terms of safety. This is slightly unfair since explosions of LIB have been extremely rare, but this issue is real and has led to replacing the lithium anode with graphite (and silicon) to avoid Li dendrites formation (which creates micro short circuits).
There is also a school of thought that LIB with the current chemistries will soon plateau in terms of energy density, hence the renewed interest in using Li metal at the anode as protection layers, for example in the form of membranes, to remedy the dendrite issues. Another similar cell architecture is called anode-free, i.e. no anode at time of charging: Li is plated upon charge and dendrites may be avoided if a solid electrolyte is used. Not everyone agrees that solid electrolytes may completely stop dendrite formation, but promising data have been circulated to evidence that this works.
Following on from the concept of solid electrolytes are solid state batteries, which are seen as the next generation alternative to Li ion. SSB could yield north of 500 Wh/kg, especially when also using Li metal anodes, but this technology is still in development. In particular, various chemistries are being considered in terms of the electrolyte: sulphide-based may have higher interfacial stability but could require care in manufacturing and operation due to the possible creation of toxic H2S. Oxide-based electrolytes are seen as being safer but some work remains to be done to yield very high C-rates and charging times. An alternative, which may result in quicker time to market, is to add a small percentage of a polymeric phase to the solid electrolyte, but not so much that the cell needs heating to 60-80°C to operate.
Ilika has over ten years’ experience developing SSB, initially at the mAh-level, for next-generation medical implanted devices and IoT sensors: this is our Stereax program which will be available at the end of 2022. Based on our knowledge of solid state materials and processes, Ilika is now firmly progressing with the development of SSB cells at Wh-level for EV and consumer electronics applications, albeit with different materials and processes than Stereax. Please contact us for more information: info@ilika.com